The following is an article from Silixa which featured in the ACG’s Caving 2022 Supplement.
Silixa shines light on mining operations by providing innovative geotechnical monitoring and imaging solutions on and below the surface
By Dr Zara Anderson and Jon Furlong, Silixa
Introduction
As a current industry leader in distributed fibre-optic sensing (DFOS) for environmental and geoscience, infrastructure, alternative energy, and oil and gas, Silixa is now focusing its lasers at the mining sector to tackle some of the industry’s most pressing challenges. Technological advances of the past decade have allowed fibre-optic investigative techniques to advance from laboratory to commercial application in a short period of time.
Some interrogation techniques, like distributed temperature sensing (DTS), have been around for decades; however, more recently, distributed acoustic sensing (DAS) and distributed strain sensing (DSS) are being adopted by industry leaders. By turning an inexpensive fibre-optic cable into a dense, distributed array of sensors, DFOS allows denser sampling of key parameters for monitoring structural integrity of tailings storage facilities (TSFs) and microseismic detection and rock mass response monitoring in the mining sector.
With a modest investment, the technology can help enhance operations while reducing risk and ultimately reducing social and economic costs. The inherent characteristic of the technology allows for a low-maintenance platform and low carbon footprint with big data deliverables for real-time analytics and AI capability.
Distributed fibre-optic sensing collects data by pulsing light rapidly down a length of fibre and recording the different backscattering phenomena. If the refractive index of the fibre is known (i.e. the speed of light in the cable), and the timing of the pulses, all recorded data backscatter to a point along the fibre (Figure 1a).
This process can turn a 50 m or 50 km length of fibre into a dense array of sample points with spatial sampling as low as
25 cm. Comprehensive spatial sampling allows for more accurate measurements and reduces the risk of incomplete measurements common with discrete sampling methods (Figure 1b). For further discussion on the technology, including how different light scattering phenomena allows for measurements of temperature, strain, and acoustics, please visit silixa.com.

Figure 1. (a) Schematic of fibre and interrogator capturing signal. (b) Benefits of distributed sensing compared with discrete sampling methods with a dip in the signal only captured on the fibre measurement.
DamPulse™: the fibre-optic sensing-based tailings dam monitoring solution
DamPulse is a comprehensive four-tier monitoring system designed for TSF operations. It enables operators to identify non-conformance in dam design or operation, and to facilitate timely maintenance and intervention before any failure occurs. Each tier of the solution can be used independently or integrated into one comprehensive platform. Figure 2 shows the sample schematic for a DamPulse monitoring system installed at a TSF.
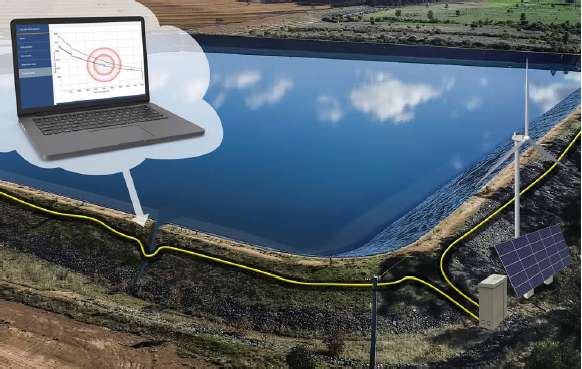
Figure 2. An illustration of how DamPulse uses fibre to monitor an entire dam in real time.
Dam breach detection is the most basic of services provided. With dam breach detection, the continuity of the cable is verified continuously. If a sudden failure occurs, the time and position of the failure is reported instantly. Distributed temperature sensing uses diurnal to seasonal variations in ambient temperature to monitor changes in seepage flow and distribution throughout the dam structure. High-resolution temperature data with fine spatial sampling is continuously acquired using Silixa’s ULTIMA™ DTS™ or XT-DTS™. In partnership with HydroResearch, Silixa has deployed this solution on over 100 embarkment dams worldwide. Real-time evaluation of both temperature and seepage information identifies areas experiencing variable flow rates, and may be susceptible to erosion – a leading cause of dam failure.
Distributed strain sensing allows for detection of small amounts of movement within the dam structure at high spatial resolutions. Silixa’s DSS monitoring system can detect deformation of the fibre-optic cable at a microstrain resolution or better, and with spatial sampling as small as 25 cm. Real-time detection of strain changes (associated with movement) in the dam can be used as a warning of unintended deformation and can assist with early decision-making and risk-mitigation strategies.
Distributed acoustic sensing turns the fibre-optic cable into a dense seismic array that measures phase, amplitude and frequency over its length with channel spacing as low as 1 m. This in effect could turn a 10-kilometre length of fibre into a seismic array like laying 10,000 one-component geophones end-to-end. These systems can record acoustic data continuously to monitor local or regional seismicity, or capture data on demand for the purpose of seismic imaging such as regional tomography or ambient noise tomography.
Rock mass response monitoring
Rock mass response monitoring with fibre is a common practice in the oil and gas industry but is at an earlier stage of adoption in mining. The rock mass response solution for mines typically focuses on DAS and DSS. DAS turns a fibre into a series of full waveform sensor, implying it is sensitive to amplitude, frequency and phase. This means that for many applications, DAS solutions act as a suitable supplement or replacement for geophones. A sample microseismic event arrival over a long borehole is shown in Figure 3 and outlines the advantages of dense spatial sampling that cannot be overstated. Geophone-based location methods typically depend on the accurate picking of primary and secondary wave arrivals, then using a time residual approach to find the location that best minimises the cumulative time between the theoretical and picked arrivals for all sensors.

Figure 3. Sample microseismic event recorded on a Carina® Sensing System. The microseismic event (a) has a lot of detail, including P-waves,
S-waves, reflected waves, and S–P conversions. Example DAS recording showing polarity (b) of an event with clear P, SH, and SV arrivals, with polarity reversals observed in the SH and P (from Baird et al. 2020).
The major shortfall of this approach is that time residuals, and therefore location errors, are high when working in a poorly defined velocity space. This is less of a concern when working in predictable, well-sequenced stratigraphy with little structure. An example would be many onshore oil field operations where the rock mass is often relatively homogenous with minimal velocity contrast. Conversely, mining velocity models are typically heterogenous with changing rock types and velocities, and with abundant structure. The dense sampling native to DAS increases hypocentre location accuracy by modelling more ray paths and minimising the effects of velocity ambiguity. Moreover, further spatial sampling of the propagating wavefield is expected to add additional accuracy to moment tensor inversions.
In some DAS recordings, the focal sphere transition is readily seen in the seismic recordings. Finally, tomographic imaging of the subsurface benefits from additional ray paths to increase the accuracy of subsurface models. Figure 4 shows ray paths modelled from two boreholes that contain geophones and DAS monitoring solutions. In this example, DAS monitoring allows for a 20-fold increase in modelled ray paths over geophones. The additional signal allows for higher-resolution spatial modelling with fewer necessary seismic points.
DFOS allows for absolute and/or dynamic strain measurements. DAS interrogators allow for dynamic strain measurements with their ability to measure very low frequencies. While geophone signals become aliased below ~10–15 Hz, DAS has a flat system response to DC, meaning it can maintain accurate measurements below 0.1 Hz. DSS systems specifically measure absolute strain by using a different light scattering phenomenon (Brillouin versus Rayleigh). Strain can be measured in boreholes, at the surface along subsidence zones or pit walls, or in the subsurface for infrastructure monitoring like tunnels, shafts and drifts. As mines are complex systems that reshape their surrounding stress conditions during development, tools that accurately quantify strain responses are important for safe operation.
To accurately measure strain, the fibre must be well coupled (e.g. for boreholes this typically means grouting). A DSS system can uniquely measure the absolute strain acting on tens of kilometres of fibre, and with a relatively low data volume. Silixa’s DSS interrogator has a resolution of ±2 με or better with temperature correction, and with a spatial resolution of 50 cm.

Figure 4. Ray paths modelled from three hypocentre locations for a two-borehole scenario in a gradually increasing velocity space. With eight geophones, a total of 24 ray paths are modelled through the rock mass. For fibre, every 1 m of cable is a geophone sensor, for a total of 160 recording channels and 480 rays total.
Why fibre-optics sensing?
DFOS is a multidisciplinary tool with a rapidly expanding userbase. While the mining industry has been underserved by this technology, adoption is beginning to accelerate due to the relatively low costs of installation and the growing range of applications. Silixa works with our mining partners to design each solution depending on the project objectives, site conditions and the desired outcome and deliverable. With a sustained demand for mineral extraction, DFOS can help us explore for new deposits in brownfield development, safely manage seismic hazard as we extend our mines deeper, and help monitoring surface operations like TSFs, open pits, and subsidence.
Zara Anderson
Silixa, Canada
John Furlong
Silixa, Canada